Researchers at Harvard’s Wyss Institute have developed an ultra-strong hydrogel adhesive inspired by the glue secreted by a common slug. The adhesive is biocompatible, flexible, and compared to the best available medical adhesives it;
1) Binds to tissues with 3-times stronger tension
2) Stretches 20-times its initial length
3) Attaches to a variety of internal body wet and moving tissue surfaces
Adhesives for internal use in humans that can bond strongly to biological tissue and surfaces can have wide ranging biomedical applications, e.g., tissue repair, drug delivery, wound dressings, and biomedical devices. However, existing adhesives each present with their problems. For example, though cyanoacrylate (Super Glue) is the strongest class of tissue adhesive it(s):
– Cytotoxic
– Not effective in adhering to wet surfaces
– Solidifies when its exposed to water
– Forms into rigid plastic
– Cannot accommodate dynamic movements of tissue
Also, nanoparticles with mussel-inspired adhesives do not adhere well to tissues, as their adhesion mainly relies on relatively weak physical interactions. Other adhesives, such as the fibrin glue and polyethylene glycol–based adhesives can form covalent bonds with tissues. But their matrix toughness and adhesions’ capabilities remain weak. Therefore, these brittle adhesives are vulnerable to debonding because of cohesive failure in the adhesive matrix.
Band-Aid® adhesive bandages are an effective tool for mitigating external bleeding from superficial skin wounds. But when surgeons need to stop internal bleeding, such a tool is yet to exist. Internal surgical glues and other materials are now often used instead of traditional wound closure techniques like stitches, staples, and clips as they reduce the patients’ time in hospital by lowering infection rates, and secondary injury/damage at the site of the internal wound.
An effective surgical glue material needs to be adaptable in diverse environments. It needs be strong, flexible, non-toxic, and able to accommodate movement in the presence tissues, blood, organs, etc. Yet there are no such adhesives currently available that possesses all of these properties. But researchers at the Wyss Institute may have discovered one. It’s this super-strong hydrogel adhesive that’s inspired by the glue secreted by a common slug. Its biocompatible, flexible, and can adhere to moving tissues even in the presence of fluid, such as blood.
A team of researchers from the Wyss Institute for Biologically Inspired Engineering and the Harvard John A. Paulson School of Engineering and Applied Sciences (SEAS) has created this super-strong “tough adhesive” that is biocompatible and binds to tissues with a strength comparable to the body’s own resilient cartilage, even when they’re wet. “The key feature of our material is the combination of a very strong adhesive force and the ability to transfer and dissipate stress, which have historically not been integrated into a single adhesive,” says corresponding author Dave Mooney, Ph.D., who is a founding Core Faculty member at the Wyss Institute and the Robert P. Pinkas Family Professor of Bioengineering at SEAS.
Lead author Jianyu Li, Ph.D. (former Postdoctoral Fellow at the Wyss Institute and now an Assistant Professor at McGill University) thought of improving medical adhesives, he found a solution in an unlikely place: the Dusky Arion (Arion subfuscus) slug. It’s common in Europe and parts of the United States, and secretes a special kind of tough sticky mucus. When threatened, the slug secretes this glue-like mucus onto the predator. The mucus is so strong and resilient it makes it difficult for the predator to pry itself away.
This super-strong adhesive’s predecessor utilized a single-layer polymer matrix with a positively charged surface. This inspired Li and his colleagues to create a double-layered hydrogel instead. It consists of an alginate-polyacrylamide-chitosan polymer matrix supporting the adhesive inner adhesive layer. that has positively-charged polymers on its surface.
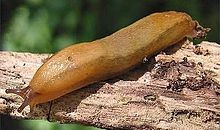
The polymers bond to tissue via three mechanisms:
1) Electrostatic attraction to negatively charged cell surfaces
2) Covalent bonding
3) Physical interpenetration
This added polymer matrix layer is thought to be as equally important to the functionality of the adhesive as inner layer. Dr. Li says, “most prior material designs have focused only on the interface between the tissue and the adhesive. Our adhesive is able to dissipate energy through its matrix layer, which enables it to deform much more before it breaks.” The design of the outer-layer matrix includes calcium ions that are bound to the hydrogel via ionic bonds. When stress is applied to the adhesive, the ionic bonds break, allowing the matrix to absorb a large amount of energy before its structure becomes compromised. In experiments, more than three-times the energy was needed to disrupt the tough adhesive’s bonding compared with other medical-grade adhesives. When the material did break, it was the hydrogel itself, and not the bond between the adhesive and the tissue, demonstrating an unprecedented level of simultaneous high adhesion strength and matrix toughness.
Credit: Video – Wyss Institute at Harvard University
The hydrogel adhesive was tested on a variety of dry and wet swine tissues including skin, cartilage, heart, artery, and liver. Results demonstrated that the adhesive bonded to all of these surfaces with significantly greater strength than other medical adhesives. Its tough adhesive properties were also demonstrated when implanted in a rat model. It also sealed a hole in a moving pig heart during tens of thousands of cycles of stretching via mechanical inflation and deflation of the heart. Further, it caused no tissue damage or adhesions to surrounding tissue when applied to a liver hemorrhage in mice.
This unprecedented hydrogel adhesive material can potentially be used in a number of biomedical and pharma applications. For example, it can be used as an internal patch which can be cut to desired sizes to be applied to various tissue surfaces and wounds of various depths. As an injectable solution or a 3d material for deeper injuries; both with the ability to cover small or large surface areas. As a drug delivery system and/or facilitator to targeted cells, tissues, and structures. “This family of adhesives may be useful in many areas of application, including tissue adhesives, wound dressings, and tissue repair.,” says co-author Adam Celiz, Ph.D., who is now a Lecturer at the Department of Bioengineering, Imperial College London. “We can make these adhesives out of biodegradable materials (such as alginates and/or chitosans), so they decompose once they’ve served their purpose. We could even combine this technology with soft robotics to make sticky robots, or with pharmaceuticals to make a new vehicle for drug delivery.”
“Nature has frequently already found elegant solutions to common problems; it’s a matter of knowing where to look and recognizing a good idea when you see one,” says Wyss Founding Director Donald Ingber, who is also the Judah Folkman Professor of Vascular Biology at Harvard Medical School and the Vascular Biology Program at Boston Children’s Hospital, as well as a Professor of Bioengineering at Harvard SEAS. “We are excited to see how this technology, inspired by a humble slug, might develop into a new technology for surgical repair and wound healing.”
This technology is available for licensing.
Original Paper: https://science.sciencemag.org/content/357/6349/378
Original Article: https://wyss.harvard.edu/technology/strong-stretchy-hydrogel-adhesives-that-can-seal-wounds-almost-anywhere-in-the-body/
Special acknowledgment to Lindsay Brownell for article excerpts: (https://wyss.harvard.edu/sticky-when-wet-strong-adhesives-for-wound-healing/)
Additional contributors to this work include co-first author Jiawei Yang, Ph.D., Research Assistant at SEAS; Qing Yang, Ph.D., Associate Professor of Environmental Science and Engineering at Huazhong University of Science and Technology; Isaac Wamala, M.D., Research Fellow at Massachusetts General Hospital; William Whyte, Research Fellow at the Wyss Institute and SEAS; Bo Ri Seo, Ph.D., Postdoctoral Fellow at the Wyss Institute and SEAS; Nikolay V. Vasilyev, M.D., Assistant Professor of Surgery and Research Scientist at Boston Children’s Hospital; Joost J. Vlassak. Ph.D., Abbott and James Lawrence Professor of Materials Engineering at SEAS; and Zhigang Suo, Ph.D., Allen E. and Marilyn M. Puckett Professor of Mechanics and Materials at SEAS.
This research was funded by the Wyss Institute at Harvard University, NSF, Materials Research Science & Engineering Centers at Harvard University, NIH, Science Foundation Ireland, Tsinghua University, as well as a Marie Curie International Outgoing Fellowship.