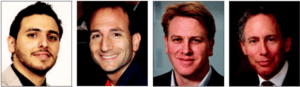
1 David H Koch Institute for Integrative Cancer Research, Massachusetts Institute of Technology, Cambridge, MA, USA
2 Department of Chemical Engineering Massachusetts Institute of Technology, Cambridge, MA, USA
3 Department of Anesthesiology, Boston Children’s Hospital, Boston, MA, USA
4 Institute for Medical Engineering and Science Massachusetts Institute of Technology, Cambridge, MA, USA
5 Harvard-MIT Division of Health Science and Technology Massachusetts Institute of Technology, Cambridge, MA, USA Email: rlanger@mit.edu (*Corresponding Author)
Introduction
As a polysaccharide, chitosan can have a large density of reactive groups and a wide range of molecular weights (Mw). Chitosan is a linear heteropolymer of N-acetyl-d-glucosamine and d-glucosamine linked by β-(1–4) glycosidic bonds (Figure 1). It is obtained by partial deacetylation of chitin, the second largest and most abundant polysaccharide in nature after cellulose. The degree of acetylation (DA) is an essential characteristic of chitin and chitosan. It represents the fraction of N-acetyl-d-glucosamine relative to the total number of units.
For many years chitosan was considered useful as a bioadhesive material because of its ability to form non-covalent bonds with biological tissues, mainly epithelia and mucous membranes. Bioadhesions formed using natural polymers have unique properties as a carrier because they can prolong residence time and, therefore, increase the absorbance of loaded drugs.1 Chitosan is hydrophilic and soluble in acidic solutions through the protonation of its amine groups. Modified and unmodified chitosan has been widely used, albeit with different molecular weights and chemical modifications, in biomedical,2,3 pharmaceutical,4 metal chelation,5,6 food additive,7 and other industrial applications.8 Chitosan is biocompatible and can be biodegraded by enzymes such as lysozymes, some lipases, and proteases.9 These properties, as well as its positive charge in physiological conditions, endow chitosan with a promising future as a biomaterial.
Nanoparticle drug delivery systems, including nanospheres, nanocapsules, nanomicelles, nanoliposomes, etc., are nanometric carriers used to deliver drugs or biomolecules by trapping active agents in their interior structures and/or adsorbing them onto their exterior surfaces.1,10–11 Presently, nanoparticles (NPs) have been widely used to deliver drugs, polypeptides, proteins, vaccines, genes, and nucleic acids. Recently, there has been increased interest in the use of NPs containing natural polysaccharides for drug delivery applications.12–13 A large number of studies have been conducted on polysaccharides and their derivatives for their potential application as NP drug delivery systems, and chitosan has been identified among the most promising candidates.14–16
The following sections focus on the leading techniques for preparation and application of chitosan NPs, as well as chemical modification methods for self-assembly structures including nanoparticles and nanomicelles.
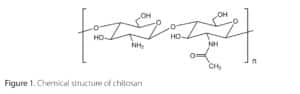
Chitosan NanoParticles Preparation Methods
Particulate chitosan structures are 3D crosslinked networks where polymeric chains are interconnected by crosslinkers. The main parameter, which determines the properties of a crosslinked NP, such as drug release and mechanical strength, is the crosslinking density.17 Depending on the desired chitosan structural characteristics, nanoparticles are prepared mainly by four mechanisms:
- Covalent crosslinking
- Ionic crosslinking
- Polyelectrolyte complexation
- Self-assembly of hydrophobically modified polysaccharide
In general, for mechanisms 1–3, chitosan NP preparation starts with the dropwise addition of the desired crosslinker to the chitosan solution with continuous stirring for 1–24 h, with or without slight heating depending on the crosslinking chemistry. However, the fourth mechanism of NP preparation, in addition to hydrophobic moiety chemistry, depends on two parameters: the percentage of hydrophobic substitution (Degree of Substitutions—DS%) and the final modified chitosan concentration (lower for nanoparticles and nanomicelles; higher within hydrogels). Table 1 summarizes and compares these four mechanisms as well as current delivery applications. Figure 2 represents illustrative photos on the crosslinked chitosan 3D structure formation highlighting internal interactions. Hydrophobic groups on chitosan confer new physicochemical properties, including the ability to self-associate in water or under sonication,18–19 to form different types of drug delivery systems (Figure 2).
Crosslinked Chitosan Nanoparticles and Chemical Modifications for Drug Delivery Applications
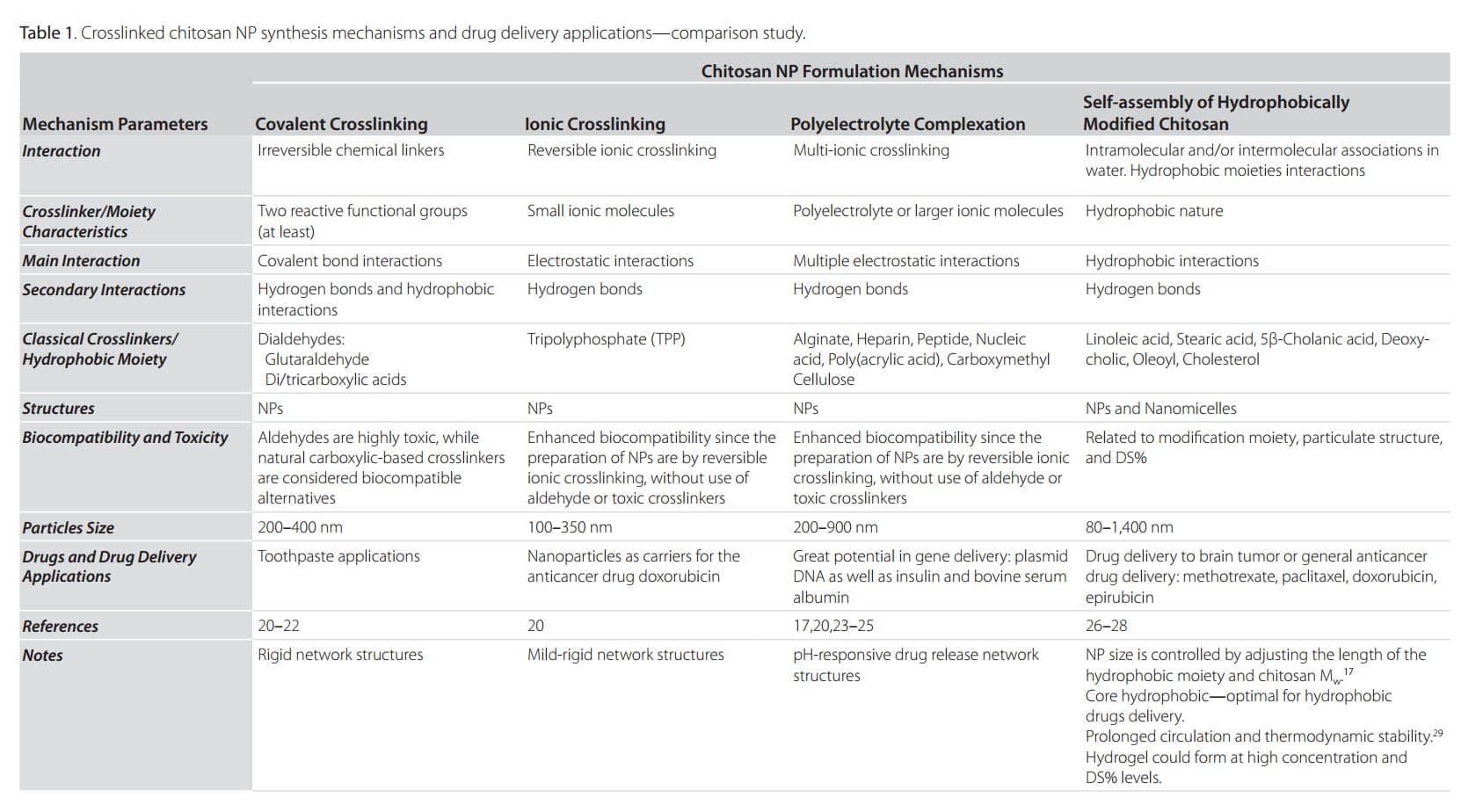
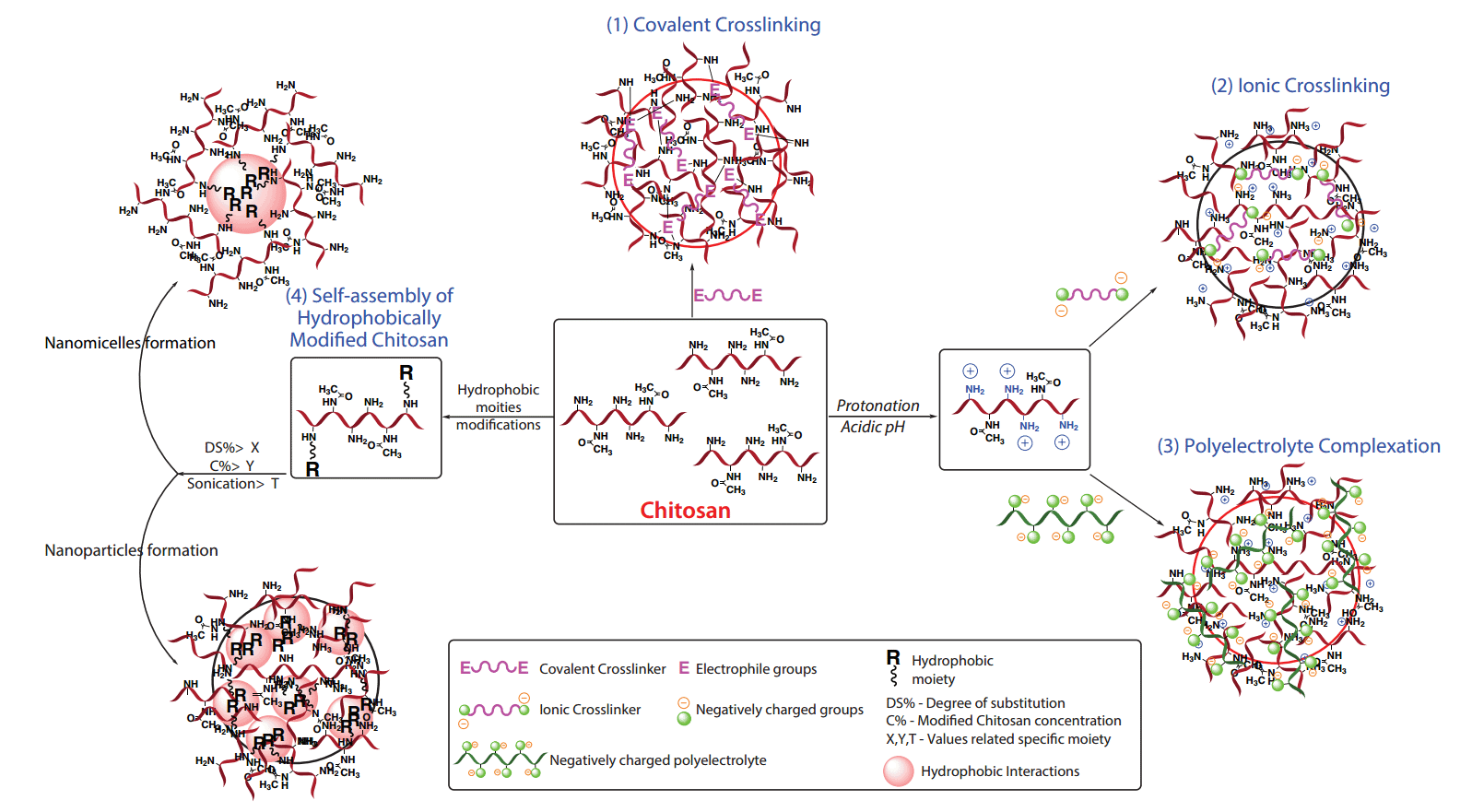
Identification of the most appropriate mechanism for chitosan nanoparticle formation can be complicated. Many factors must be considered that may affect the rational design of nanoparticulate delivery systems, including drug nature and loading capacity, delivery duration, chitosan Mw, NP shape and size, targeting site, and biocompatibility. On the other hand, several factors also influence the crosslinking reaction such as the characteristics and chemical structure of the crosslinker itself. In covalent crosslinking, for example, the most crucial factor is the crosslinker size/length; in ionic crosslinking, the global charge of both the crosslinker and the polysaccharide are dominant. Additionally, unlike covalently crosslinked NPs, ionically crosslinked particles are generally pH sensitive, a desired trait for drug delivery purposes.
Chitosan Modifications: NPs and Nanomicelles
In addition to Mw diversity, chitosan has a variety of reactive groups, including hydroxyl and amino groups (Figure 1), which allow for the possibility of chemical modification to obtain amphiphilic properties.1 This gives it a new or improved property. Chitosan, chemically modified through the grafting of hydrophobic groups, undergoes intra and/or inter-molecular hydrophobic interactions. Amphiphilic properties allow it to self-associate in aqueous solution, leading to different kinds of drug delivery systems such as nanomicelles, nanoparticles, microspheres,30 liposomes,31 and hydrogels (Figure 2. Mechanism 4). Modified chitosans are of great interest for the development of various controlled-release systems.
Since the amine group of chitosan is more reactive than the hydroxyl groups, all the research describing the formation of amphiphilic chitosan have been based on the chemical grafting of hydrophobic groups on the amine functional group by N-acylation reactions.32 The following section reviews experimental procedures for the chemical modification of chitosan based on N-acylation and current applications in drug delivery systems, as listed in Table 1 (self-assembly part) and Table 2.
Delivery of hydrophobic molecules and proteins has always been an issue due to poor bioavailability after administration. Micelle carrier systems can improve drug solubility and stability as well as help overcome toxicity and immunogenicity problems. It is well known the hydrophobic core of the micelles provides a reservoir for loading water-insoluble drugs. By grafting hydrophobic moieties to the polysaccharide backbone, self-assembled micelles can be readily formed in aqueous solution. Aggregation of amphiphilic polymers is controlled by the balance between the interaction of the hydrophobic groups and the hydrophilic chains. The critical aggregation concentration (CAC) is the concentration at which the polymer aggregation starts.
It was found that a chitosan EDC-mediated modification with stearic acid, linoleic acid, deoxycholic acid, and 5β-cholanic acid tends to self-aggregate to form nanomicelles at very low CAC (0.01–0.06 mg/mL). 33–36 Amphiphilic chitosan-based micelles were used to encapsulate doxorubicin,37 paclitaxel,27,34,38 ibuprofen,39 and the amphiphilic adriamycin.40 Furthermore, hydrophilic peptides, proteins, and nucleic acids41,42 can be adsorbed onto chitosan-based micelles.
Concluding Remarks
This short review summarizes the recent research on chitosan and its amphiphilic derivatives, including experimental chemical modifications, the mechanisms of nano-structure fabrication, and their drug delivery system applications. Special attention is increasingly focused on modified chitosan with amphiphilic properties because of unique properties such as excellent biocompatibility and biodegradability, non-toxicity, and bioadhesive properties. Self-assembly of amphiphilic chitosan provides further promise for drug delivery systems since several properties such as size, surface charge, loading efficiency, stability, and biodistribution can be altered for a particular application. Chitosan-based nanomicelle systems have been found to improve delivery of hydrophobic drugs and proteins, increase stability, and exhibit controllable drug release properties, biocompatibility, and improved targeting for specific applications—all of which are of increasing interest for clinical application.
Chitosan Nanoparticles by Covalent or Ionic Crosslinking
Chitosan nanoparticles can be formed by a variety of methods including emulsion, ionic gelation, reverse micellar methods, or self-assembly. The example method given here uses the simplest approach to form either chemical or ionic crosslinked chitosan nanoparticles. This method utilizes dropwise addition of a crosslinker to a solution of chitosan under constant stirring.
1. Prepare chitosan solution: Dissolve chitosan at 0.1–1 wt% in 1–3% acetic acid, once dissolved adjust pH to 4.7.
2. Prepare crosslinker solution:
a) For Ionic gelation: dissolve crosslinker (Tripolyphosphate (TPP), dextran sulphate, etc.) in deionized water at 0.1 wt% to 0.5 wt%.
b) For covalent crosslinking: adjust crosslinker concentration (example: 1–3% dialdehyde or di/tricarboxylic acid).
3. Slowly add crosslinker solution (1 mL) dropwise into Chitosan solution (3 mL) at room temperature with constant stirring.
4. Allow nanoparticles to stabilize by a 30 min incubation at room temperature.
5. Collect nanoparticles by centrifugation at 13,000 × g at 10 °C for 30 min, and resuspend nanoparticles in aqueous solution.
6. To incorporate drug, add drug to crosslinker aqueous solution prior to addition to the chitosan solution.
Note: Chitosan and crosslinker concentration need to be optimized for desired particle size and encapsulation efficiency.
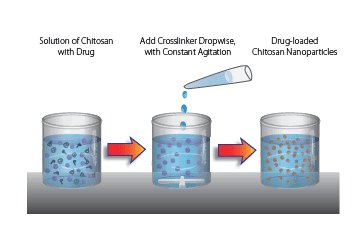