Chitosan oligosaccharide / Chito-oligosaccharide (COS) is an oligomer of β-(1➔4)-linked d-glucosamine. COS can be prepared from the deacetylation and hydrolysis of chitin and chitosan, which is commonly found in the exoskeletons of arthropods, including crustaceans and insects, and cell walls of fungi.
Introduction
Agriculture faces challenges to find effective, sustainable, and ecologically sound solutions for the enhancement of crop productivity. There is an emerging consensus that chitosan oligosaccharide (COS) can play an important role in making agriculture more efficient in terms of increasing plant, crop, and fruit yields and environmental sustainability. According to numerous reports, water-soluble chitosan oligosaccharides have been shown to induce various plant defense-related cellular responses.1
Chitosan oligosaccharide is a natural Biofertilizer, Biopesticide, and Biostimulant (Plant Immune Elicitor). It induces broad-spectrum antifungal, antibacterial, and antiviral activity against pathogens and pests and enhances plant growth and productivity for significant increases in crop yield.

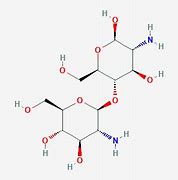
COS Capabilities for Crop and Plant Productivity
- Activates Plant Defense Responses Vs. Fungal, Bacterial, Viral Diseases & Environment Stress – Enhances plants’ defense response for disease resistance vs. bacterial, viral, and fungal pathogens and pests – Stimulates plants’ biochemical pathways that strengthen plant structure and act against fungal pathogens – Induces plant immune reactions & plant self-defenses that increase resistance against environment stress (heat, cold, drought, etc.)
- Improves Soil Structure
– Improves soil condition and restores soil ecology
– Stimulates root growth
– Soil Treatment that prevents harmful pathogens and nematodes
– Elicits the growth and activity of useful microbes, such as chitinase, chitosanase, l-3-glucanase, rhizobacteria, etc. - Promotes Plant Growth and Enhances Yield – Seed germination and seedling growth – Root, stalk, and leaf growth
– Fruit growth
– Mineral absorption accelerant
- Seed Pre-Treatment – Improves seed growth, vigor, and germination
– Stimulates seed immune and growth mechanisms to improve disease resistance and enhance growth rates
Chitosan Oligosaccharide Studies
Improved Strawberry Fruit Quality (2)
This study investigated the pre-harvest treatment effect of COS on the quality of strawberries (Fragaria × ananassa cv. qingxiang).
The results indicate that the pre-harvest spraying of COS had positive effects on the quality improvement of strawberries, such as fruit firmness, viscosity, lignin, vitamin C, total phenol, and antioxidant activity. In addition, COS also increased the cell wall content and suppressed the expression of genes involved in the ethylene signaling pathway, which reduced the softness and increased the shelf life of fruit.
These results imply that COS may not only improve strawberry quality but also have a positive effect on extending its shelf life. Combined with its well-known plant defense induction activity, COS could be used as an eco-friendly, nontoxic substitute substance for traditional pesticides in fruit crops.
Reduces Disease on Tomatoes (3)
Chitosan Oligosaccharide significantly reduced disease lesion size on tomato fruits when the tomato plants were pretreated with 1.0% or 2.5% (w/v) COS solution 10 days before being inoculated with Colletotrichum sp.
Potent Plant Immunity Elicitor (4)
Chitosan oligomers are known elicitors of plant defense mechanisms. In this work, chitosan oligosaccharides of different degrees of polymerization and degrees of acetylation were prepared and characterized by matrix-assisted laser desorption ionization time-of-flight mass spectrometry. The effect of the degree of polymerization (DP), degree of acetylation (DDA%), and concentration of these chitosan oligosaccharides on defense activation in Arabidopsis thaliana suspension-cultured cells were studied. Our study results show that fully deacetylated chitosan oligosaccharides (chitosan oligomers) induce, depending on their DP and concentration, phenylalanine ammonia-lyase (PAL) activation, H2O2 synthesis, and cell death in A. thaliana cell suspensions. The progressive reacetylation of the chitosan oligomer elicitors progressively impaired their ability to enhance H2O2 accumulation and cell death but did not affect the activation of PAL. COS has been considered a potent plant immunity elicitor and used on different plants such as Arabidopsis, tobacco, and grapevine.
Inhibition of Pathogen Growth by Induction of Plant Resistance (5)
Enzymatic degradation of chitosan polymer with Pectinex Ultra SPL was used to obtain derivatives with biological potential as protective agents against Phytophthora parasitica nicotianae (Ppn) in tobacco plants. The 24 h hydrolysate showed the highest Ppn antipathogenic activity and the chitosan native polymer was the lowest. The in vitro growth inhibition of several Phytophthora parasitica strains by two chitosans of different DA was compared. While less acetylated chitosan (DA 1%) fully inhibited three P. parasitica strains at the doses of 500 and 1000 mg/l the second polymer (DA 36.5%) never completely inhibited such strains.
When comparing two polymers of similar molecular weight and different DA, again the highest antipathogenic activity was for the less acetylated polymer. However, degraded chitosan always showed the highest pathogen growth inhibition. Additionally, a bioassay in tobacco seedlings to test plant protection against Ppn by foliar application demonstrated that partially acetylated chitosan and its hydrolysate induced systemic resistance and higher levels of glucanase activity than less acetylated chitosan.
Similarly, when treatments were applied as seeds coating before planting, about 46% of plant protection was obtained using chitosan hydrolysate. It was concluded that, while less acetylated and degraded chitosan is better for direct inhibition of pathogen growth, partially acetylated and degraded chitosan are suitable to protect tobacco against P. parasitica by systemic induction of plant resistance.
Increases Wheat Yield (6)
Chitosan Oligosaccharide (COS) increases wheat field mainly via increased spike number, grains per spike, and total grain yield were increased by spraying COS at tillering stage of wheat.
COS seed dressing and foliar spraying showed diverse effects on wheat production.
The specific wheat cultivars played an important role in the effects of COS: irrigated cultivars were more sensitive to COS than the rainfed cultivar in this study.
COS acts as a growth promoter in wheat production, and a possible strategy for applying COS in wheat production is proposed.
Promotes Quality and Antioxidant Activity of Strawberry (7)
Composites coated with chitosan and chitosan derivatives demonstrate positive effects in maintaining higher concentrations of total phenolics and anthocyanins. The coatings also maintained lower activities of cell wall degrading enzymes.
It’s concluded that the chitosan and chitosan derivative coatings improved the quality of strawberries and that they are useful for extending the shelf-life and preserving the quality of strawberry fruit.
Promotes polyphenol Content in Greek Oregano (8)
The induction of resistance to Sclerotinia sclerotiorum in Brassica napus L. var. Huyou 15 by Chitosan Oligosaccharide (COS) was studied. Even though COS (50 μg mL−1) did not affect radial growth of this pathogen in vitro, it reduced the disease symptoms in vivo relative to control plants. The disease control rates were 25.8%, 41.4%, 57.1%, 68.7%, and 48.8% with COS pre-treatment 0, 24, 48, 72, and 96 h before S. sclerotiorum inoculation, respectively.
Specific binding of COS to B. napus epidermis cells was validated by competition experiments. Simultaneously, it was observed that COS induced bursts of cytosolic Ca2+, nitric oxide (NO), and hydrogen peroxide (H2O2). NO and H2O2 inhibitors were used to prove the interaction between NO and H2O2. Furthermore, treatments of B. napus with NO and H2O2 inhibitors reduced the induction effect of the jasmonic acid–ethylene (JA/ET) signaling marker BnPDF1.2 by COS, indicating that NO and H2O2 participate in COS-induced JA/ET signaling. In conclusion, this article provides a comprehensive study of the effect and mechanism of COS-induced resistance to S. sclerotiorum in B. napus.
Improves vitamin and polyphenol content in cherries (9)
The objective of this research was to investigate the effect of treatment with chitosan and Chitooligosaccharide (COS) on vitamin C and polyphenols contents in cherries during refrigerated storage. The treatment with chitosan and chitooligosaccharides promotes vitamin C synthesis in cherries. Higher values of anthocyanin content for cherries treated with chitosan and chitooligosaccharide compared with anthocyanin content in control cherries were found after 7 days of storage at 4 °C. Total phenols content in cherries increased after storage for 7 days at 4 °C.
Chitosan Oligosaccharide an Immunity Elicitor in Plants (10)
Excerpts of a Case Study
Chitosan oligosaccharide (COS) is an effective plant immunity elicitor; however, its induction mechanism in plants is complex. In this case study, COS mechanisms’ effects on Arabidopsis–Pseudomonas syringae pv. tomato DC3000 (hereafter called DC3000) was investigated.
COS is effective in inducing resistance to DC3000 in Arabidopsis. Results of the study demonstrate that treatment with COS 3 days before DC3000 inoculation provided the most effective resistance. Disease severity in jar1 (jasmonic acid [JA]-deficient mutant), NahG, and sid2 (salicylic acid [SA]-deficient mutants) suggest both the SA and JA pathways are required for the Arabidopsis response to DC3000. COS pre-treatment induced resistance in wild type (WT), jar1, and also, although to a lesser degree, in NahG and sid2 plants, implying that the SA and JA pathways play redundant roles in COS-induced resistance to DC3000. In COS-pretreated plants, the expression of genes related to the SA pathway (PR1, PR2, and PR5) and SA content increased in both WT and jar1. Moreover, the expression of genes related to the JA pathway (PDF1.2 and VSP2) and JA content both increased in WT and NahG. In conclusion, COS induces resistance to DC3000 in Arabidopsis by activating both SA- and JA-mediated pathways, although SA and JA pathways play redundant roles in this COS-induced resistance.
The interaction of Arabidopsis thaliana and Pseudomonas syringae pv. tomato (DC3000) has been used for many years as a typical plant pathosystem to study plant defense response (Jovanovic et al. 2017; Katagiri et al. 2002; Liu et al. 2015; Naseem et al. 2014).
When pathogens attack plants, various signaling pathways are activated, including the salicylic acid (SA)- and the jasmonic acid/ethylene (JA/ET)-mediated signaling pathways (Kouzai et al. 2018; Zhang et al. 2017). In both basal and induced resistance in plants, the SA and JA/ET pathways influence each other by acting in synergistic and antagonistic ways (Betsuyaku et al. 2018; Koornneef and Pieterse 2008; Zhang et al. 2015). According to previous studies, plants that overaccumulate SA or have been treated with exogenous SA are more resistant to DC3000, suggesting that SA plays an essential role in Arabidopsis resistance to DC3000 (Todesco et al. 2010; Velásquez et al. 2017; Wilson et al. 2017). Generally, SA and JA pathways work in an antagonistic way; however, some studies have found that both SA- and JA-mediated pathways are required for Arabidopsis resistance to DC3000. During DC3000 infection, both the SA and JA pathway-related genes in Arabidopsis are upregulated, leading to SA and JA accumulation in infected leaves (Betsuyaku et al. 2018; Hossain et al. 2008; Khare et al. 2016; Kloek et al. 2001).
In plant-pathogen interactions, microbe-associated molecular patterns (MAMPs) such as chitin and flagellin are perceived by plant receptors that activate plant innate immunity to defend against pathogen invasion (Poncini et al. 2017). Chitosan oligosaccharide (COS) is an effective MAMP that induces plant innate immunity (Yin et al. 2010, 2016). According to previous studies, COS serves as a potent immunity elicitor in many plants, including tobacco (Zhang et al. 2012), camellia (Li and Zhu 2013), wheat (Wang et al. 2015), oilseed rape (Yin et al. 2013), tomato (Zhang and Chen 2009), soybean (Valdés-López et al. 2014), Arabidopsis (Jia et al. 2016), and rice (Yang et al. 2017).
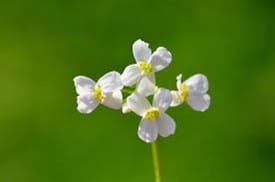
Chitosan’s induction mechanism on fungi is revealed by inserting itself into the minor groove of DNA and activating DNA damage responses to potentiate plant immunity (Hadwiger 2015; Hadwiger and Tanaka 2017). This signal perception mode is quite different from chitin oligomers, which are recognized by receptors at the plasma membrane by affecting transcription factors without directly acting on DNA (Desaki et al. 2018; Hadwiger and Tanaka 2017; Squeglia et al. 2017). Recently, a work has revealed that fluorescence-labeled COS are enriched on the wheat leaf cell surface, and several candidates that have the potential to be COS receptors were found (Liu et al. 2018). Thus, besides acting on DNA directly, COS receptors on plant plasma membrane maybe also exist.
Although the COS signal perception mechanism is by DNA damage responses or receptor recognition, the downstream signal transduction pathways are similar. As a broad-spectrum plant immunity elicitor, COS activates different signaling pathways for defense against pathogen invasion. Previous studies have demonstrated that COS treatment enhances the expression of JA-related genes in Brassica napus (Yin et al. 2006) and increases JA content in tomato (Doares et al. 1995), rice (Rakwal et al. 2002), and rapeseed (Yin et al. 2013). These results reveal that COS enhances plant immunity mainly through the JA-mediated pathway. However, when COS-treated Arabidopsis or tobacco plants are infected with the Tobacco mosaic virus (TMV), only the SA-mediated pathway is activated (Jia et al. 2016; Yafei et al. 2009). Similar results were observed in COS-pretreated rice, where infection with the Southern rice black-streaked dwarf virus (SRBSDV) dramatically increased PR1 transcription level (Yang et al. 2017). Thus, because of the complexity of the COS-induced immune signaling network, the topic requires further research.
In this study, Arabidopsis–P. syringae pv. tomato DC3000 interaction was used to further reveal the complex mechanisms of COS-induced immunity. The involvement of SA and JA-mediated pathway in COS-induced resistance to DC3000 in Arabidopsis were investigated by using SA- or JA-deficient mutants, including jar1 (JA pathway-deficient mutant), NahG, and sid2 (SA pathway-deficient mutants).
Discussion
However, in Arabidopsis-DC3000 interaction, both SA and JA signaling pathways were activated; thus, they may work in independent or cooperative manners without obvious antagonism. (Betsuyaku et al. (2018) found that, although the SA and JA pathways are both activated in the Arabidopsis-DC3000 interaction, they are active in different domains around the infection site. While SA accumulates in the cells directly around DC3000 infection sites, the JA pathway is activated in cells surrounding SA-active cells (Betsuyaku et al. 2018).
DC3000 resistance was more obviously impaired in NahG and sid2 than jar1, revealing the more critical role of the SA-mediated pathway than JA pathway in Arabidopsis defense to DC3000. According to previous studies, virulence systems of DC3000 usually promote infection by targeting the JA signaling pathway. For example, DC3000 is able to synthesize the polyketide toxin coronatine, which structurally mimics JA, interfering with the JA-mediated signaling pathway (Staswick and Tiryaki 2004; Xin and He 2013; Zhao et al. 2003). This may be responsible for the major role of the SA signaling pathway in limiting DC3000 infection in Arabidopsis.
COS pretreatment activated both SA- and JA-mediated pathways in WT Arabidoposis, which all worked positively in Arabidopsis defense to DC3000. However, SA and JA pathways play redundant roles in COS-induced resistance to DC3000 in SA or JA pathway–deficient mutants due to the highly activated JA or SA pathway after COS pretreatment. This induction effect of COS that activates different signaling pathways under different situations makes COS work effectively in multiple plant-pathogen interactions. This phenomenon also appeared in other elicitor-treated plants, according to previous studies, such as plant growth-promoting fungus Penicillium simplicissimum GP17-2 (Hossain et al. 2007) and Phoma sp. strain GS8-1 (Hossain et al. 2008). GP17-2 or GS8-1 pretreatment was fully protective against DC3000 in jar1, similar to WT, and also worked effectively in NahG and npr1, although to a lesser degree. Furthermore, treatment with GP17-2 or GS8-1 resulted in enhanced expression of SA- and JA-related genes and activated both SA and JA pathways in WT, just like COS-induced resistance.
Generally, the SA signaling pathway is associated with biotroph resistance, whereas the JA/ET signaling pathway is involved in defending against necrotrophs (Robert-Seilaniantz et al. 2007). COS-induced resistance in the Arabidopsis-DC3000 (hemibiotrophic pathogen) interaction is mainly achieved by activating both SA- and JA-mediated pathways. This is quite different from Arabidopsis-TMV (biotrophic pathogen) interactions, since COS only activates the SA signaling pathway (Jia et al. 2016). Further, in tomato, rice, and rapeseed, COS pretreatment activates the JA-dependent signaling pathway, which results in resistance to necrotrophic pathogens (Doares et al. 1995; Rakwal et al. 2002; Yin et al. 2013). Based on these results, COS pretreatment activates different defense pathways in different plants under infection by different pathogens, activating one or both the SA-dependent and JA-dependent, signaling pathways. Thus, COS functions as an effective and broad-spectrum plant immunity elicitor in multiple plant-pathogen interactions.
The broad-spectrum disease control effects demonstrated by COS pretreatment in different plant-pathogen interactions are ubiquitous among plant immunity elicitors. For example, the well-studied pathogen-associated molecular pattern flg22 was found to induce resistance in Arabidopsis against DC3000 by activating both SA- and JA-dependent signaling pathways (Navarro et al. 2004; Wang et al. 2018). Additionally, flg22 induced resistance to Golovinomyces cichoracearum in Arabidopsis by activating the SA signaling pathway (Lu et al. 2015). In addition to flg22, other plant immunity regulators, such as harpin, Bacillus cereus AR156, and plant growth-promoting fungi also showed similar induction responses (Chuang et al. 2014; Hossain et al. 2008; Jiang et al. 2016; Nie et al. 2017; Wang et al. 2018).
Certainly, signaling pathways in addition to SA and JA pathways may also be involved in COS-induced resistance.
We are grateful to authors of this study Xiaochen Jia, Haihong Zeng, Wenxia Wang, Fuyun Zhang, and Heng YinXiaochen Jia, et., Chitosan Oligosaccharide Induces Resistance to Pseudomonas syringae pv. tomato DC3000 in Arabidopsis thaliana by Activating Both Salicylic Acid– and Jasmonic Acid–Mediated Pathways. Molecular Plant-Microbe Interactions 2018 31:12, 1271-1279 [Ref]
The excerpts taken from these studies are not originally written by ChitoLytic, Inc. They are used here in this publication for only the purposes of distribution of what we/ChitoLytic believe to be useful information for its readership and customers.
We are grateful to authors of this study Xiaochen Jia, Haihong Zeng, Wenxia Wang, Fuyun Zhang, and Heng YinXiaochen Jia, et., Chitosan Oligosaccharide Induces Resistance to Pseudomonas syringae pv. tomato DC3000 in Arabidopsis thaliana by Activating Both Salicylic Acid– and Jasmonic Acid–Mediated Pathways. Molecular Plant-Microbe Interactions 2018 31:12, 1271-1279 [Ref]
The excerpts taken from these studies are not originally written by ChitoLytic, Inc. They are used here in this publication for only the purposes of distribution of what we/ChitoLytic believe to be useful information for its readership and customers.
References
- Hadwiger LA. Multiple effects of chitosan on plant systems: solid science or hype. Plant Sci. 2013 Jul;208:42-9. [PubMed]
- He Y, Bose SK, Wang W, Jia X, Lu H, Yin H. Pre-Harvest Treatment of Chitosan Oligosaccharides Improved Strawberry Fruit Quality. Int J Mol Sci. 2018 Jul 27;19(8):2194. [Free Full-Text]
- Munoz, Z.; Moret, A.; Garces, S. Assessment of chitosan for inhibition of Colletotrichum sp. on tomatoes and
Crop. Prot. 2009, 28, 36–40. [CrossRef] - Cabrera, J.C.; Messiaen, J.; Cambier, P.; Van Cutsem, P. Size, acetylation and concentration of chitooligosaccharide elicitors determine the switch from defence involving PAL activation to cell death and water peroxide production in Arabidopsis cell suspensions. Plant 2006, 127, 44–56. [CrossRef]
- Falcon, A.B.; Cabrera, J.C.; Costales, D.; Ramırez, M.A.; Cabrera, G.; Toledo, V.; Martınez-Tellez, M.A. The
effect of size and acetylation degree of chitosan derivatives on tobacco plant protection against Phytophthora
parasitica nicotianae. World J. Microbiol. Biotechnol. 2008, 24, 103–112. [CrossRef] - Wang, M.; Chen, Y.; Zhang, R.; Wang, W.; Zhao, X.; Du, Y.; Yin, H. Effects of chitosan oligosaccharides on the yield components and production quality of different wheat cultivars (Triticum aestivum ) in Northwest China. Field Crops Res. 2015, 172, 11–20. [CrossRef]
- Gol, N.B.; Patel, P.R.; Rao, T.V.R. Improvement of quality and shelf-life of strawberries with edible coatings enriched with chitosan. Postharvest Biol. Technol. 2013, 85, 185–195. [CrossRef]
- Yin, H.; Li, Y.; Zhang, H.Y.; Wang, W.X.; Lu, H.; Grevsen, K.; Zhao, X.; Du, Y. Chitosan oligosaccharides triggered innate immunity contributes to oilseed rape resistance against Sclerotinia sclerotiorum. J. Plant Sci. 2013, 174, 722–732. [CrossRef]
- Kerch, G.; Sabovics, M.; Kruma, Z.; Kampuse, S.; Straumite, E. Effect of chitosan and chitooligosaccharide on vitamin C and polyphenols contents in cherries and strawberries during refrigerated storage. Food Res. Technol. 2011, 233, 351–358. [CrossRef]