University of Genova (Italy) Researchers maybe the first to demonstrate the ability of pure chitosan (a natural linear polysaccharide) to assist in neuronal cell attachment and functional neuronal network development. This exciting research can be a pivotal step towards achieving low-cost biomimetic culture systems; Brain-On-A-Chip Experimental Model. In turn, this can have important implications in a wide range of applications, such as neuropharmacology, toxicology, and regenerative medicine, to name a few.1,2
Introduction
The availability of 3D biomimetic in vitro neuronal networks of mammalian neurons represents a pivotal step for the development of brain-on-a-chip experimental models to study neuronal (dys)functions and particularly neuronal connectivity. The use of hydrogel-based scaffolds for 3D cell cultures has been extensively studied in recent years. However, only limited work on biomimetic 3D neuronal cultures has been carried out to date.
It’s on this background University of Genova, researchers from the Departments. of Informatics, Bioengineering, Robotics and System Engineering investigated the use of chitosan in producing microbeads 3D scaffold to be combined with primary neuronal cells. These chitosan microbeads were characterized by optical and atomic force microscopies. The cell/scaffold interaction was extensively characterized transmission electron microscopy and by immunocytochemistry confocal microscopy. Finally, a preliminary electrophysiological characterization by micro-electrode arrays was carried out.
The physico-chemical characteristics of the extracellular matrix (ECM) play a fundamental role in regulating physiological cellular processes and in a variety of pathological situations.3,4 Therefore, it’s apparent that cellular organization in 3D is vital in studying biological functions.5,6 To date, most in vitro functional studies have been performed using oversimplified traditional monolayer cultures. However recently, a growing number of research groups have been focusing on developing cellular models which better mimics the in vivo microenvironment.7,8 This approach is thought to be essential in gaining important information on pathological processes, such as in cancer, where cell-to-cell and cell-microenvironment interactions play an important role.9
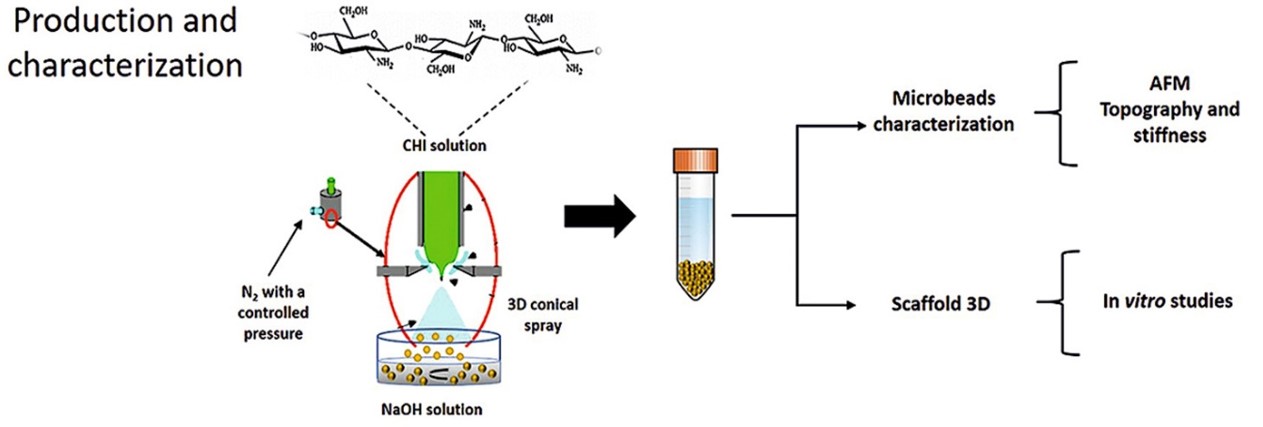
Characterization of Chitosan Microbeads
In this research, the use of Chitosan Microbeads to dynamically support 3D functional neuronal cultures was explored. Chitosan was the biopolymer selected for its biocompatibility, biodegradability, and low cost.10 Additionally, literature reports that the positive charges of primary amines onto the polymer backbone favor the electrostatic interaction with the negatively charged cell membranes, promoting cell adhesion and growth.11,12,13,14
In general, the stiffness, porosity, and electrostatic charge of the scaffold aligns with native neuritic development and extension. In addition the stiffness of 1% Chitosan Microbeads was determined to be comparable to that of brain tissue. A difference in the stiffness between 1% and 2% Chitosan Microbeads was observed. This difference could be attributed to the increase in the concentration of chitosan, which corresponds to an increase in the density of the polymeric chains. Therefore, higher ionic interactions between the chitosan chains appears to render the scaffold to be relatively predictable due the increase in the concentration of chitosan from 1% to 2%.15 Furthermore, as seen in the results, the lower concentration of chitosan in the Chitosan Microbeads is the higher its water content which in turn confers a decrease in scaffold stiffness.16 However, this effect is not considered to be the major factor in the rheology of the scaffold, especially related to its stiffness. Whereas, the main mechanism for the determination of the scaffold’s stiffness is the increase in the ionic interactions in the biopolymer complex.
Atomic Force Microscopies (AFM) revealed darker areas on the scaffold’s surface, which may represent holes whose apparent dimensions align with data obtained by Transmission Electron Microscopy (TEM) within this research. These characteristics combined with the bioaffinity of chitosan due to the presence of primary amines, is a significant contributor to the formation of a dense neuronal network onto Chitosan Microbeads.
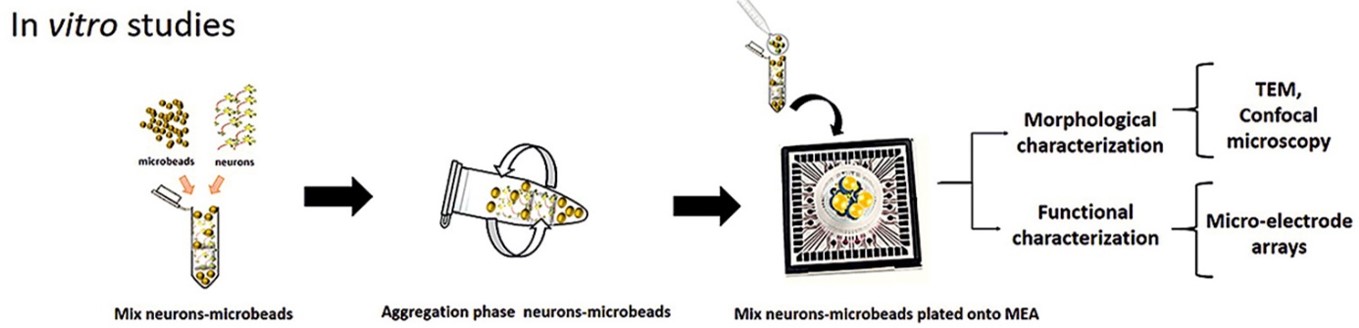
Conclusion
The availability of 3D culture platforms designed to mimic different tissues towards the development of organ-on-a-chip, is expected to have a significant impact the study of physiological and pathological processes, drug screening, and in toxicity assays. Therefore, the development of 3D in vitro models is a fundamental step in engineering and tailoring of a 3D matrix which contains relevant and sufficient chemical and mechanical signals in order to support specific cell phenotypes.
Synthetic and natural hydrogels have been used to develop 3D models for soft tissues due to their hydrophilicity, biocompatibility, biodegradability, and tunable microporosity. It is known that these 3D interconnected networks of neuronal cells are very useful in studying in vitro models for neuronal (dys)functions and connectivity for applications ranging from basic neuroscience to drug screening.14,15
In this research, Chitosan Microbeads based 3D scaffolds were optimized and adapted to be integrated onto planar MEAs to study and better understand the functional properties of biomimetic 3D hippocampal networks. Chitosan Microbeads both treated and untreated with adhesion factors were tested. Whereas, both proved to be reliable supports, able to sustain the neuronal populations during the growth in a 3D space. In addition, the Chitosan Microbeads provided both a morphological and structural development of a functional network. This research demonstrated that the neuronal network itself was responsible for the assembly and the stabilization of the 3D chitosan based structure. In conclusion, chitosan seems to be a promising scaffolding-support for developing 3D neuronal networks towards the design and implementation of brain-on-a-chip microsystems.
With all this, the Researchers have recently demonstrated that 3D hippocampal networks, made by self-assembled glass microbeads as scaffold, and coupled to micro-electrode arrays (MEAs), represent a suitable in vitro model for neurophysiological studies alternative and complementary to the older 2D neuronal network models. Matrix stiffness and composition are the most critical properties of the 3D system as they can influence growth dynamics, synaptic density, and electrophysiological activity of the neuronal network.
In conclusion, a soft porous hydrogel microbeads platform is developed which mimics the physico-chemical characteristics of the Extra Cellular Matrix (ECM), for the growth of 3D neuronal networks. Chitosan microbeads based scaffolds were synthesized and optimized in order to be integrated onto planar MEAs to study and better understand the functional properties of biomimetic 3D hippocampal networks. Chitosan microbeads both treated and untreated with adhesion factors were tested. Both proved to be reliable and able to sustain the neuronal population during the growth in a 3D space. In addition, the chitosan microbeads proved to provide both a morphological and structural development of a functional network. Finally, the Researchers demonstrated that the neuronal network itself was responsible for the assembly and the stabilization of the 3D chitosan based structure. In conclusion, Chitosan appears to be a promising scaffolding-support for developing 3D neuronal networks towards the design and implementation of brain-on-a-chip microsystems.
References
-
S.S. Lim, D.C. Foo, Simulation and scale-up study for a chitosaneTiO2 nanotubes scaffold production, Food Bioprod. Process. 106 (2017) 108e116. [PubMed]
-
I. Hamed, et al., Industrial applications of crustacean by-products (chitin, chitosan, and chitooligosaccharides): a review, Trend. Food Sci. Technol. 48 (2016) 40e50. [PubMed] [Cross Ref]
-
T. Dvir, et al., Nanotechnological strategies for engineering complex tissues, Nat. Nanotechnol. 6 (1) (2011) 13e22.
-
E. Cukierman, et al., Taking cell-matrix adhesions to the third dimension, Science 294 (5547) (2001) 1708e1712.
-
B.A. Justice, N.A. Badr, R.A. Felder, 3D cell culture opens new dimensions in cell-based assays, Drug Discov. Today 14 (1e2) (2009) 102e107.
-
L.G. Griffith, M.A. Swartz, Capturing complex 3D tissue physiology in vitro, Nat. Rev. Mol. Cell Biol. 7 (3) (2006) 211e224.
-
F. Pampaloni, E.G. Reynaud, E.H. Stelzer, The third dimension bridges the gap between cell culture and live tissue, Nat. Rev. Mol. Cell Biol. 8 (10) (2007) 839e845.
-
D.B. Kolesky, et al., 3D bioprinting of vascularized, heterogeneous cell-laden tissue constructs, Adv. Mater. 26 (19) (2014) 3124e3130.
-
K.H. Benam, et al., Engineered in vitro disease models, Annu. Rev. Pathol. 10 (2015) 195e262.
-
D.B. Kolesky, et al., 3D bioprinting of vascularized, heterogeneous cell-laden tissue constructs, Adv. Mater. 26 (19) (2014) 3124e3130.).
-
C.M. Valmikinathan, et al., Photocrosslinkable chitosan based hydrogels for neural tissue engineering, Soft Matter. 8 (6) (2012) 1964e1976.
-
Z. Cao, R.J. Gilbert, W. He, Simple agarose-chitosan gel composite system for enhanced neuronal growth in three dimensions, Biomacromolecules 10 (10) (2009) 2954e2959.
-
V.I. Scanga, et al., Biomaterials for neural-tissue engineering d chitosan supports the survival, migration, and differentiation of adult-derived neural stem and progenitor cells, Can. J. Chem. 88 (3) (2010) 277e287.
-
M. Prasitsilp, et al., Cellular responses to chitosan in vitro: the importance of deacetylation, J. Mater Sci. Mater Med. 11 (12) (2000) 773e778.
-
Dabiri, Seyed Mohammad Hossein, et al., New in-situ synthetized hydrogel composite based on alginate and brushite as a potential pH sensitive drug delivery system, Carbohydr. Polym. 177 (2017) 324e333.
-
Seung G. Lee, et al., Molecular dynamics simulation study of P (VP-co-HEMA) hydrogels: effect of water content on equilibrium structures and mechanical properties, Biomaterials 30.30 (2009) 6130e6141.)